Research Summary: Alternative Stable States In Large Shallow Lakes
01Department of Aquatic Ecology, Netherlands Institute of Ecology (NIOO-KNAW), P.O. Box 50, 6700 AB Wageningen, The Netherlands
2Department of Aquatic Ecology and Water Quality Management, Wageningen University, The Netherlands
At the end of spring 2007 the drinking water station near Wuxi (southeast China) reports smelly drinking water and needs to stop its production (Guo, 2007; Qin et al., 2010). As a result, millions of people are without drinking water (Qin et al., 2010). The cause of the smell is found in a large algal bloom in the north of Lake Taihu (2338km2) persisting for several weeks (Paerl et al., 2011). However, these problems do not appear to be temporary as the blooms became more frequent and longer lasting in the following years, and even prevails today (Figure 1) (Chen et al., 2003; Duan et al., 2009).
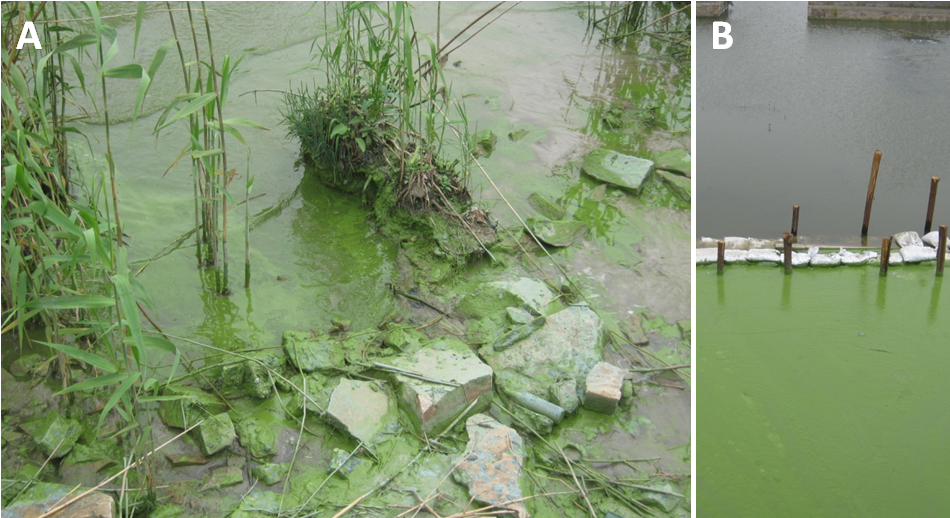
Figure 1. (A) Algal growth in Taihu: blooms accumulate at the shores. (B) Dams are used in an attempt to keep algae from the channels connected to Taihu (Credit: ABG Janssen, 2013)
Algal blooms like in Taihu are an increasing worldwide problem as a result of eutrophication. Increasing nutrient loads cause catastrophic shifts in lakes from macrophyte to phytoplankton dominance (Scheffer et al., 2001). Restoration from the eutrophic to oligotrophic state is often not straightforward due to hysteresis (Figure 2) (Scheffer et al., 1993). As result of hysteresis the trajectory of eutrophication is different from the trajectory of oligotrophication, a phenomena better known as alternative stable states. Alternative stable states have repeatedly been shown in small lakes like Lake Veluwe in the Netherlands (30 km2) (Meijer and Hosper, 1997) and the gravel pit lakes in England (< 1 km2)(Wright and Phillips, 1992). Whether alternative stable states also exist in large shallow lakes is less clear, however. In our review paper on alternative stable states in large shallow lakes this intriguing question is discussed based on lake size, spatial heterogeneity and internal lake connectivity (Janssen et al., 2014).
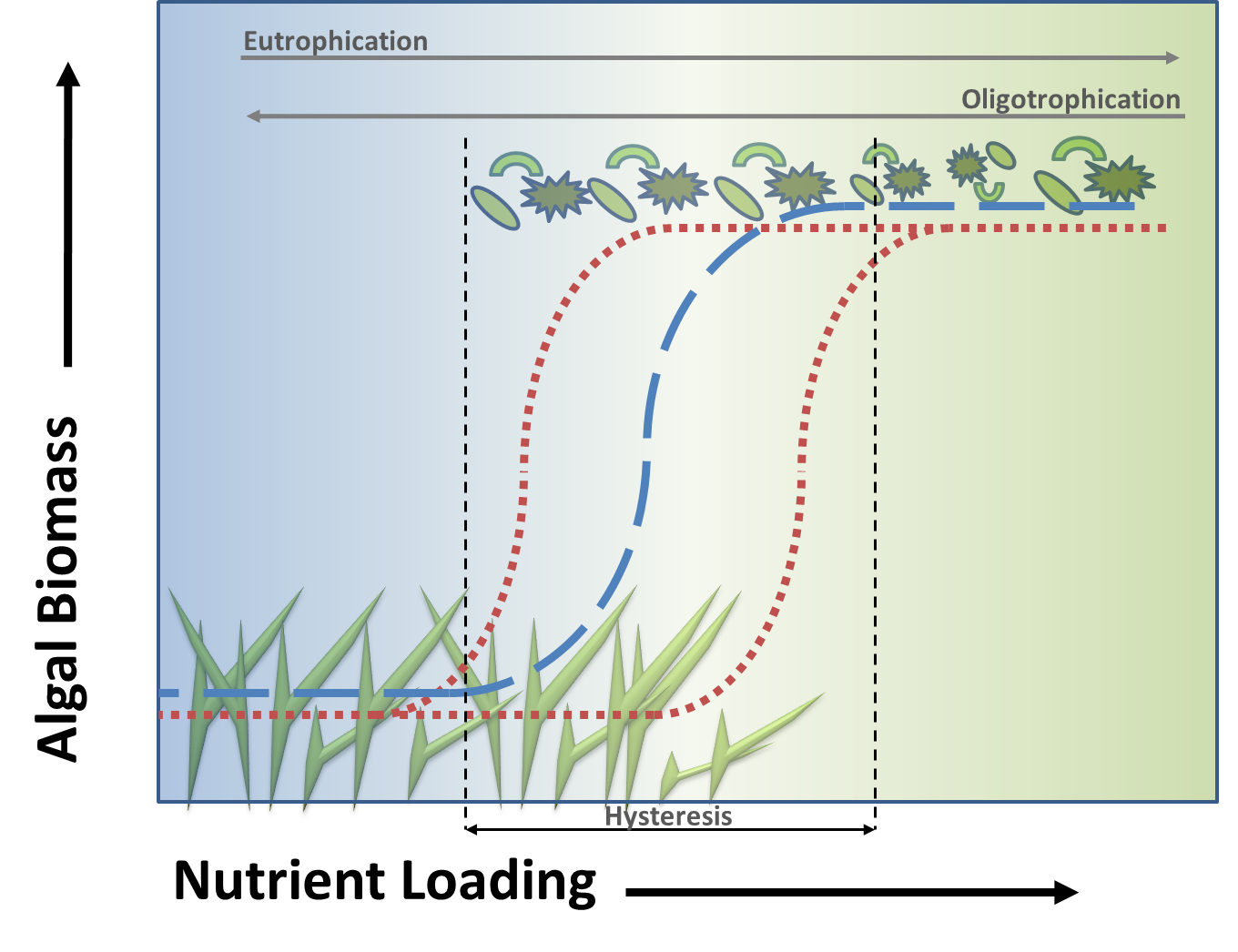
Figure 2. Lakes are often shown to respond non-linearly to eutrophication (blue dashed) and in many cases hystereses have been assumed (orange dotted). Hysteresis is nonlinear behavior where the state of a lake does not only depend on its present input but also on the prior state. As a result, two stable states could occur given the same conditions (see area of hysteresis). (Credit: Annette Janssen)
Theory
With increasing lake size, the potential for macrophyte presence decreases, here referred to as the size effect (Figure 3, ①). Indeed larger lakes have a longer fetch, allowing the wind to generate higher waves that destruct macrophytes and cause a high turbidity (Janse et al., 2008; Jeppesen et al., 2007). Additionally, larger lakes tend to be deeper which further negatively influences the light climate for macrophytes (Bohacs et al., 2003; Søndergaard et al., 2005).
Despite the size effect, macrophytes do occur and even flourish in large lakes, though they are often restricted to specific regions of the lake (Janssen et al., 2014). As a result of spatial heterogeneity (Figure 3, ②) some parts of the lake are more suited for macrophyte growth than others. The lake can for example vary in depth or fetch. The areas that are shallow and have a low fetch are then more suitable for macrophytes than deep parts with long fetch (Janse et al., 2008).
However, macrophyte presence itself does not prove alternative stable states’ existence in large shallow lakes. Positive feedbacks between macrophytes and algae need to be strong enough to exhibit hysteresis (Scheffer et al., 1993). The internal connectivity within a lake is an important factor that affects the positive feedbacks (Figure 3, ③). If, for instance, the macrophyte suitable areas are mostly isolated, they could be responding like separate small lakes. On the other hand, if the connectivity is high, they could influence other parts of the lake by introducing clear water or, vice versa, other parts of the lake could transfer more turbid water to the macrophyte suitable areas (Hilt et al., 2011).
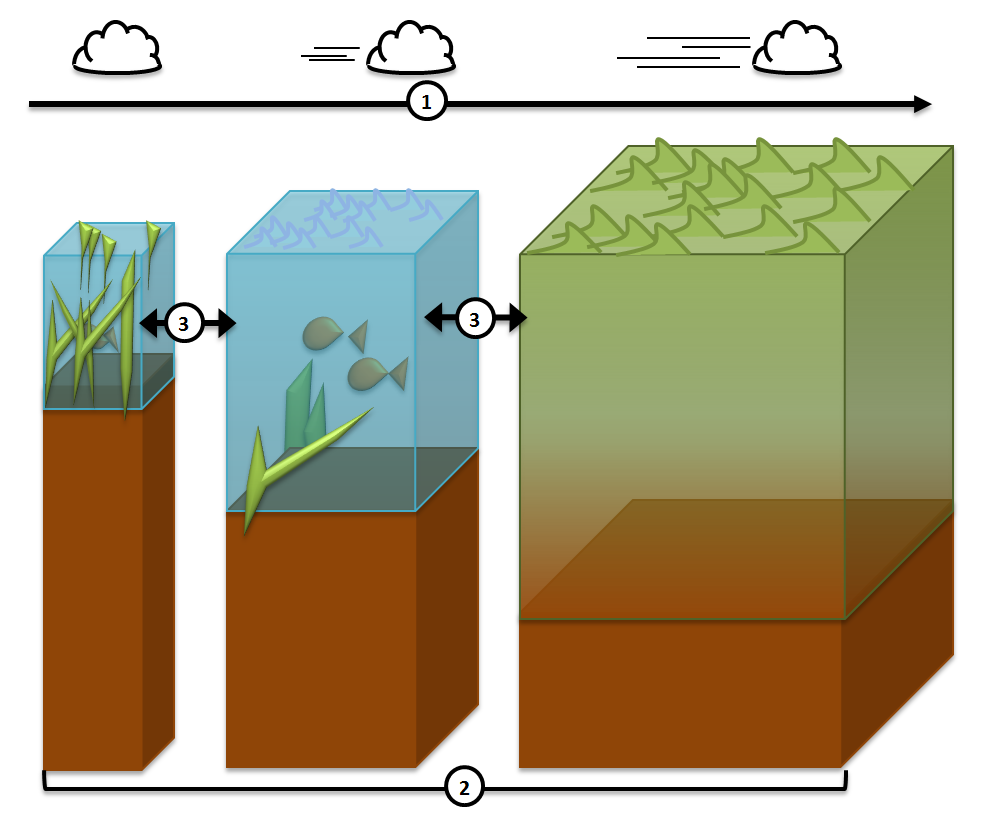
Figure 3. As lake size increases, macrophytes’ potential decreases ①. Due to heterogeneity, parts of lakes can be suitable for macrophytes ②. Depending on internal connectivity, lake parts may develop individually or affect each other ③. (Credit: Annette Janssen)
Method
With the ecosystem model PCLake, multiple bifurcation analyses have been performed using the standard parameter set (Janse et al., 2010; Janse et al., 2008). Therefore, the model has been run for different nutrient loadings ranging from oligotrophic to eutrophic and back to identify whether the lake shows hysteresis (Figure 2, orange dotted line) or not (Figure 2, blue dashed line). The bifurcation analysis has been repeated for multiple lake types differing in fetch and depth.
Result
The multiple bifurcation analyses are a first estimation on which lakes might have alternative stable states and which do not. The model outcome has been compared with data from literature. This comparison between model and data shows agreement for lakes that are particularly suitable for macrophyte growth mainly because of their shallowness (Figure 4A, ①). For instance, Lake Istokpoga (113 km2, USA, Nr. 1) is overgrown with macrophytes and multiple attempts to remove them had only temporal effects. Other lakes are suggested by the model to have alternative stable states (Figure 4A, ②) as for example Lake Apopka (125 km2, USA, Nr. 4) where literature suggested hysteresis as well. Finally there are lakes that have, according to the model, too large a fetch or are too deep to be dominated by macrophytes (Figure 4A, ③). Indeed many of these lakes are partly free of macrophytes, however most of these lakes are not completely bare. However, the effects of spatial heterogeneity are not taken into account yet. As an example, when spatial heterogeneity is taken into account for Lake Taihu, a different picture may be seen (frequency distribution, Figure 4B). This illustrates that parts of the lake are suitable for macrophyte growth, whether or not with presence of alternative stable states and other parts will exhibit the phytoplankton dominated state. While this analysis ignores the internal connectivity, its effect can be logically deduced. With a large internal connectivity, lakes will act more homogenous and therefore the area of alternative stable states will be most likely more confined.
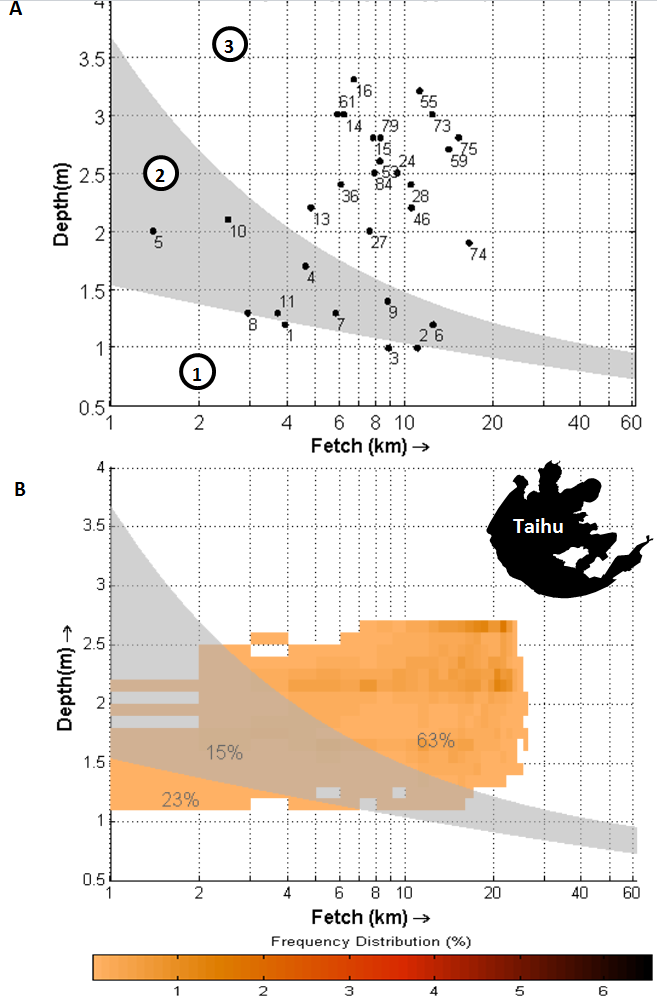
Figure 4. (A) Result from the bifurcation analysis. Lakes located in areas ① and ③ are suggested by the model to lack alternative stable states. Lakes located in the gray area denoted by ② are suggested by the model to have alternative stable states. Numbers relate to lakes listed in table 1 in Janssen et al. (2014). (B) Frequency distribution for Taihu. Darker colors mean a higher occurrence of the combination of fetch and depth in Taihu. For more detailed information, see Janssen et al. (2014). (Credit: Annette Janssen)
Discussion And Conclusion
To evaluate the presence of alternative stable states of large lakes it is important to look beyond the size effect of lakes and also take the spatial heterogeneity and internal connectivity into account. Under specific conditions, large shallow lakes may exhibit (spatial restricted) alternative stable states.
Full study published in the Journal of Great Lakes Research, December 2014, Vol. 40, Issue 4, p. 813–826.
References
- Bohacs K.M., Carroll A.R., Neal J.E. 2003. Lessons from large lake systems-Thresholds, nonlinearity, and strange attractors. Special papers-Geological Society of America;75-90.
- Chen Y.W., Qin B.Q., Teubner K., Dokulil M.T. 2003. Long-term dynamics of phytoplankton assemblages: Microcystis-domination in Lake Taihu, a large shallow lake in China. Journal of Plankton Research;25;445-53.
- Duan H., Ma R., Xu X., Kong F., Zhang S., Kong W., Hao J., Shang L. 2009. Two-decade reconstruction of algal blooms in China’s Lake Taihu. Environmental science & technology;43;3522-8.
- Guo L. 2007. Doing battle with the green monster of Taihu Lake. Science;317;1166-.
- Hilt S., Köhler J., Kozerski H.P., Van Nes E.H., Scheffer M. 2011. Abrupt regime shifts in space and time along rivers and connected lake systems. Oikos;120;766-75.
- Janse J., Scheffer M., Lijklema L., Van Liere L., Sloot J., Mooij W. 2010. Estimating the critical phosphorus loading of shallow lakes with the ecosystem model PCLake: Sensitivity, calibration and uncertainty. Ecological Modelling;221;654-65.
- Janse J.H., De Senerpont Domis L.N., Scheffer M., Lijklema L., Van Liere L., Klinge M., Mooij W.M. 2008. Critical phosphorus loading of different types of shallow lakes and the consequences for management estimated with the ecosystem model PCLake. Limnologica-Ecology and Management of Inland Waters;38;203-19.
- Janssen A.B.G., Teurlincx S., An S., Janse J.H., Paerl H.W., Mooij W.M. 2014. Alternative stable states in large shallow lakes? Journal of Great Lakes Research;40;813-26.
- Jeppesen E., Meerhoff M., Jacobsen B., Hansen R., Søndergaard M., Jensen J., Lauridsen T., Mazzeo N., Branco C.W.C. 2007. Restoration of shallow lakes by nutrient control and biomanipulation—the successful strategy varies with lake size and climate. Hydrobiologia;581;269-85.
- Meijer M.-L., Hosper H. 1997. Effects of biomanipulation in the large and shallow Lake Wolderwijd, The Netherlands. Hydrobiologia;342;335-49.
- Paerl H.W., Xu H., McCarthy M.J., Zhu G., Qin B., Li Y., Gardner W.S. 2011. Controlling harmful cyanobacterial blooms in a hyper-eutrophic lake (Lake Taihu, China): The need for a dual nutrient (N & P) management strategy. Water research;45;1973-83.
- Qin B.Q., Zhu G.W., Gao G., Zhang Y.L., Li W., Paerl H.W., Carmichael W.W. 2010. A drinking water crisis in Lake Taihu, China: linkage to climatic variability and lake management. Environmental management;45;105-12.
- Scheffer M., Carpenter S., Foley J.A., Folke C., Walker B. 2001. Catastrophic shifts in ecosystems. Nature;413;591-6.
- Scheffer M., Hosper S., Meijer M., Moss B., Jeppesen E. 1993. Alternative equilibria in shallow lakes. Trends in Ecology & Evolution;8;275-9.
- Søndergaard M., Jeppesen E., Jensen J.P. 2005. Pond or lake: does it make any difference? Archiv für Hydrobiologie;162;143-65.
- Wright R., Phillips V. 1992. Changes in the aquatic vegetation of two gravel pit lakes after reducing the fish population density. Aquatic Botany;43;43-9.
Featured Image: Lake Taihu. (Credit: Wikimedia Commons User Cocowind123 via Creative Commons 3.0)